INTRODUCTION
Soil salinity is one of the main factors affecting crop production worldwide (Machado and Serralheiro, 2017). More than 1 billion ha worldwide would be affected by this phenomenon (Ivushkin et al., 2019). Soil salt build-up can have a natural origin (primary salinization) or be the result of human activity (secondary salinization) (Yadav et al., 2011). In the latter case, the main cause of soil salinization is a combination of irrigation with water high in dissolved salts and poor drainage(Singh, 2021). It is estimated that 3 ha of agricultural land is lost every minute due to salinity worldwide (Okur and Örçen, 2020). Given that irrigation, on average, doubles crop production and there is an increased demand for food crops (Tian et al., 2021), it is likely improbable that this practice would be abandoned (Cuevas et al., 2019). Although there are practices that can reduce the risk of soil salinization, it is very difficult to cultivate in such soils, which determines, in many cases, their abandonment (Litalien and Zeeb, 2020).
In plants, salt stress affects different physiological and metabolic processes, depending on its severity and duration. A high content of salts in the soil solution reduces the osmotic potential and thus the ability of plants to acquire water (Machado and Serralheiro, 2017) which is known as the ´osmotic effect of salinity´ (Yadav et al., 2011). Negative impacts of low osmotic potential include reduced turgor, stomatal closure with the consequent reduced photosynthesis (Saffan, 2008), and inhibition of growth (Isayenkov and Maathuis, 2019). Ion toxicity is another negative effect of salinity and its main consequence is an increased generation of reactive oxygen species (ROS) (Parihar et al., 2015). Excessive accumulation of ROS affects various cellular processes through nucleic acid damage, protein oxidation, membrane lipid oxidation and chlorophyll degradation (Ahanger et al., 2017; Gill and Tuteja, 2010).
Melon (Cucumis melo L.) is an important horticultural crop with a worldwide production of 28 million tons (FAOSTAT, 2023). Soil salinity is the main abiotic factor that drastically affects melon production worldwide (Wu et al., 2019). Salinity negatively impacts many physiological processes (Akrami and Arzani, 2018; Castañares and Bouzo, 2019) and plant growth (Hniličková et al., 2019; Ibrarullah et al., 2019), which will eventually reduce fruit quality and yield (Huang et al., 2012). The greatest sensitivity to this stress occurs in early stages (Pinheiro et al., 2019) and the impact is lower if it takes place during fruit set (54–56 days after planting) and fruit growth (69-71 days after planting) (Sivritepe et al., 2005).
Proline is an amino acid that accumulates in plants in response to different types of environmental stress (high salinity, drought, high light and UV irradiation, heavy metals, oxidative, etc.) (Szabados and Savoure, 2010). Under saline or osmotic stress, proline plays an important role in the process of osmotic adjustment (Hmidi et al., 2018), subcellular structure and protein stabilization (Kaur and Asthir, 2015), and ROS neutralization (Hayat et al., 2012). Nevertheless, the proline biosynthetic pathway requires high consumption of reductants (NADH and NADPH) (Kaur and Asthir, 2015). The biosynthesis of each proline molecule consumes 30 ATP molecules (Zhang and Becker, 2015). However, exogenous application of proline has been reported to enhance the adaptive response under salt stress or drought. For example, Bhusan et al. (2016) foliar applied proline to rice (Oryza sativa L.) plants growing under saline stress and registered an increase in growth. Similar results were obtained by Freitas et al. (2019), working with sorghum (Sorghum bicolor L.). Under drought stress conditions, EL-Bauome et al. (2022) reported an increase in vegetative growth and chlorophyll content of cauliflower (Brassica oleracea var. botrytis L.) plants.
The aim of this work was to evaluate the effect of exogenous application of proline on the performance of melon seedlings under salt stress.
MATERIALS AND METHODS
Plant material
Cantaloupe melon (Cucumis melo L., var. catalupensis, cv. ‘Planter’s Jumbo’) seeds (Bonanza Seeds, California, USA) were used.
Growth conditions
Seeds were germinated in trays filled with vermiculite in a growth chamber at 60% RH, 16 h light duration, 400 µmol-2s-1 light intensity and day/night temperatures of 25/18 °C. After first leaf emergence, seedlings were transplanted to 1000 cm3 pots filled with perlite and watered with Hoagland’s nutrient solution (Hoagland and Arnon, 1950) with 2.0 dS m-1 electrical conductivity (EC). After the emergence of the second leaf, plants were divided into four groups of 20 plants each and irrigated once with 25 mL of 0, 10, 50 and 100 mM proline solutions. Additionally, all plants were manually watered with Hoagland’s nutrient solution, and 60 mM NaCl to reach 8.0 dS m-1 EC. Irrigation was applied once a day and until the substrate reached container capacity. The EC was adopted because it has been reported that at this salinity level crop yield is reduced approximately by 50% (Huang et al., 2012). The EC value was monitored with a portable conductivity meter daily.(Hanna model HI98304, Italy).
Proline content determination
Every 72 h and for 30 days, five plants per treatment were randomly selected and the last expanded leaf of the main stem was extracted to measure proline content, according to the protocol proposed by Bates et al. (1973). A sample of 300 mg fresh weight (FW) was homogenized in 10 mL of 3% aqueous sulfosalicylic acid solution and the homogenate was passed through a filter paper (Whatman No. 1). Then, 2 mL of filtrate was reacted with 2 mL of 0.2% acid ninhydrin and 2 mL of concentrated glacial acetic acid in a test tube for 1 h at 100 °C. The rreaction was stopped on ice for 15 min. The mixture was extracted with 4 mL of toluene for 20 s with a vortex. The absorption of the upper phase was measured by spectrophotometry (Shimadzu UV-1800, Japan) at 520 nm. Proline concentration was calculated using a calibration curve and expressed as µmol proline g-1 FW.
Chlorophyll determination
Leaf chlorophyll content was estimated using a portable chlorophyll reader (SPAD-502 Konica Minolta, Japan). The results were expressed in SPAD units.
Growth parameters
Ten plants from each treatment were harvested 30 days after treatment and main stem length and leaf number were measured. Stem length was obtained by measuring the extension between the insertion of the cotyledons and the apical end. The number of leaves was determined by the direct counting of the expanded leaves.
Statistical analysis
The experiment had a completely randomized design. Data were analyzed via one-way analysis of variance (ANOVA), followed by Tukey’s test at 0.05 confidence level, with Infostat statistical software (Di Rienzo et al., 2011).
RESULTS
Periodic measurements of proline content for different treatments (0, 5, 10 and 20 mM) evidenced a higher level for the 10 and 20 mM treatments at 4 days after the application of this amino acid. In contrast, for the 5 mM dose and the control treatment, the highest proline content was measured at 16 days, although this content only reached a third of the levels of the other treatments at day 4 (fig. 1).
Chlorophyll content only indicated a meaningful difference for 10 mM treatment, in which a significantly higher value was measured at 4, 20 and 28 days (fig. 2).
When comparing the response of growth parameters 30 days after treatments, a greater main stem length (fig. 3 A) and number of leaves (fig. 3 B) were recorded in 10 and 20 mM treatments.
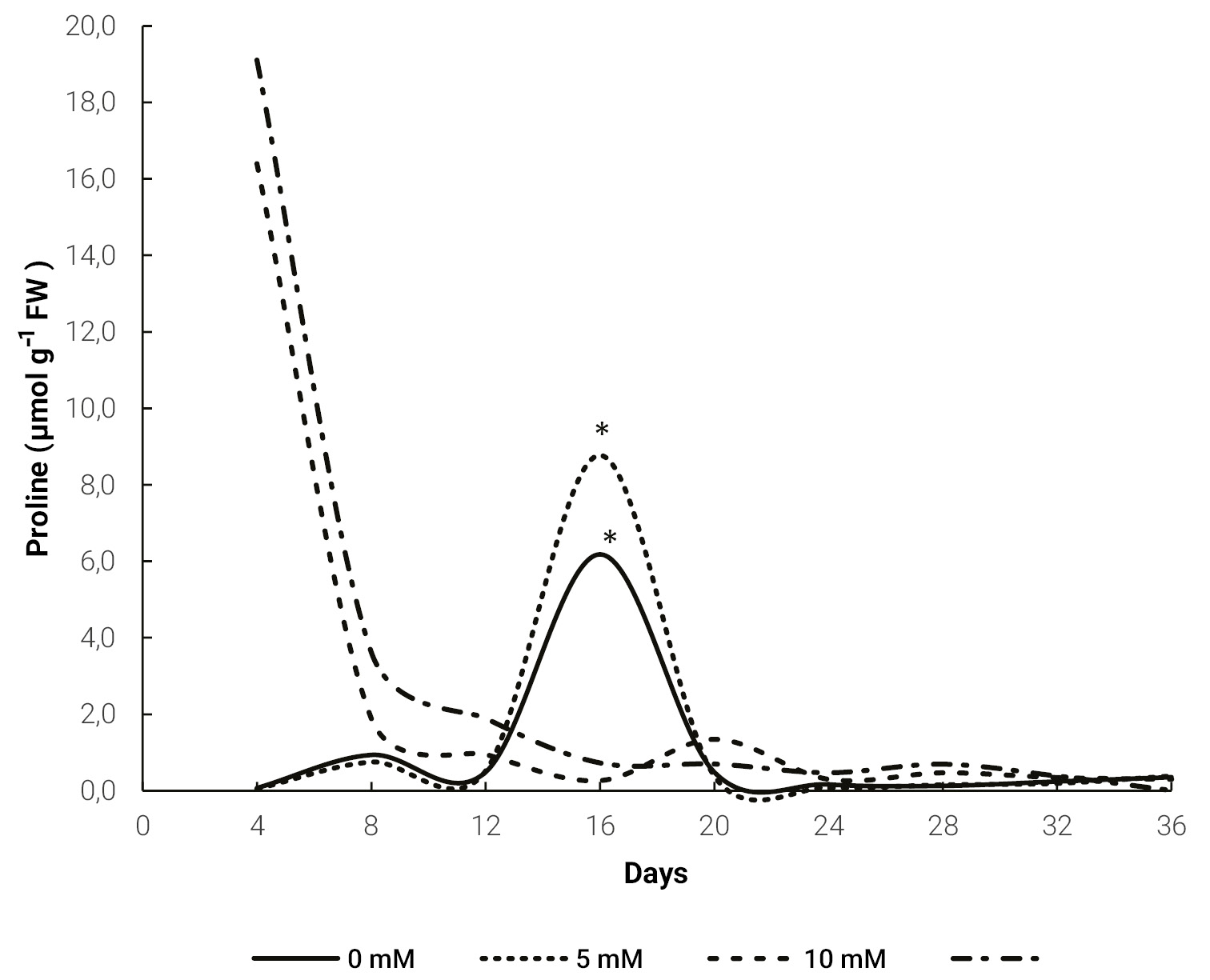
Figure 1.Evolution of proline content in Melon leaves for different doses of exogenous proline. The * indicates a significant difference (p ≤ 0.05) for the same day, obtained by Tukey’s test.
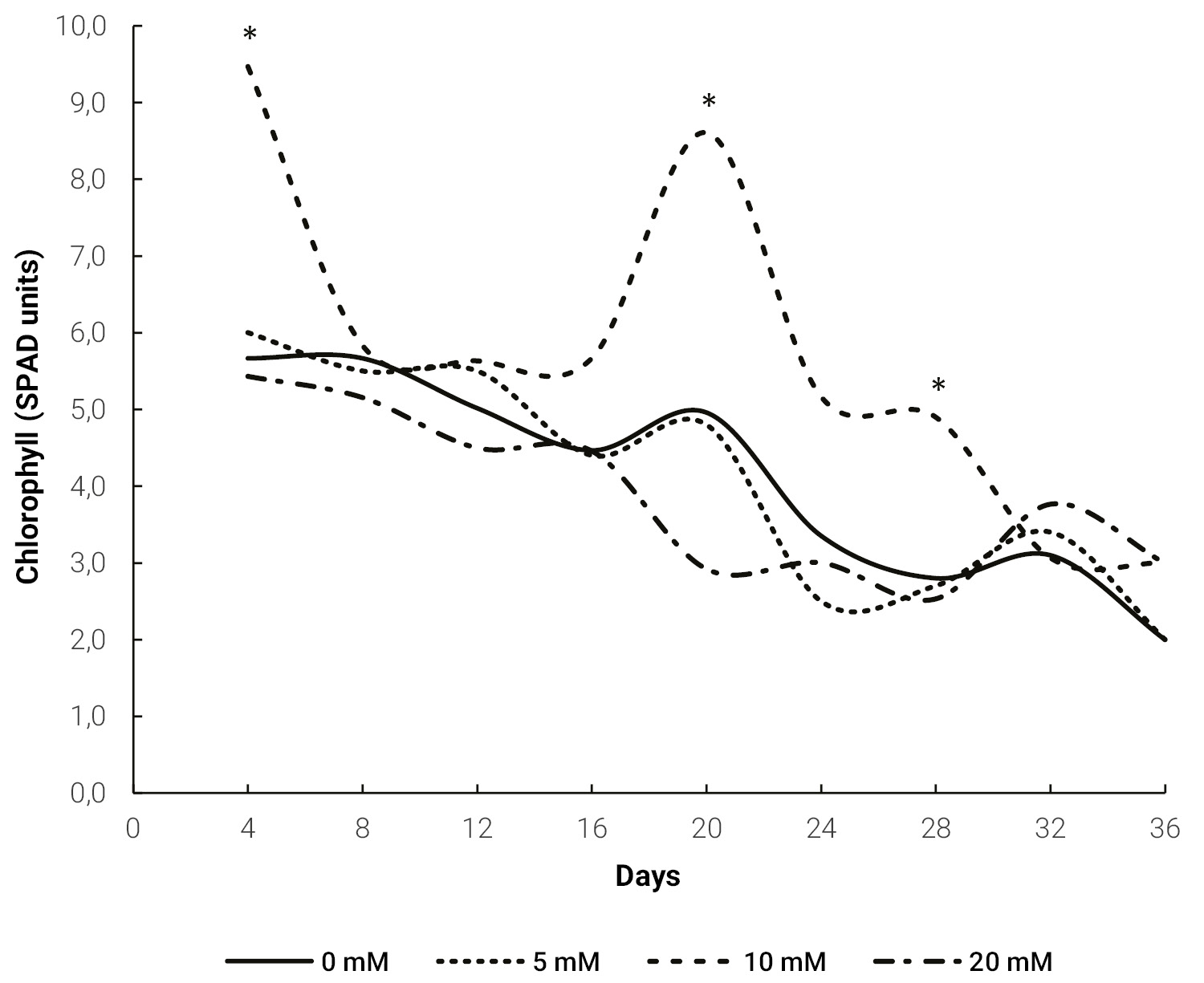
Figure 2.Evolution of chlorophyll content in Melon leaves for different doses of exogenous proline. The * indicates a significant difference (p ≤ 0.05) for the same day, obtained by Tukey’s test.
DISCUSSION
Saline stress induced an increase in proline content in plants (fig. 1). The 10 and 20 mM treatments tripled proline content, compared to untreated plants, which is generally associated with increase of tolerance (El Moukhtari et al., 2020; Hoque et al., 2007). In addition, these high values were observed 4 days after proline application. This allows us to conclude that exogenous proline applications could increase plant tolerance to saline stress and allows an earlier response. There are no previous studies analyzing the evolution of proline content in plants under stress. However, the increase in proline content in plants treated with exogenous proline and growing under such conditions has been reported in sorghum (Sorghum bicolor L.) (de Freitas et al., 2019), maize (Zea mays L.) (de Freitas et al., 2018) and bean (Phaseolus vulgaris L.) (Aggarwal et al., 2011).
In our experiments, the increase in salt stress tolerance is evident in both growth parameters evaluated, main stem length and leaf number, which were positively affected at 10 and 20 mM exogenous proline treatments (fig. 3). The improvement of plant growth under different stress situations and proline application has been reported in several crops, such as sunflower (Helianthus annuus L.) (Iqbal et al., 2014), olive (Olea europaea L.) (Aliniaeifard et al., 2016), mustard (Brassica juncea L.) (Wani et al., 2016) and eggplant (Solanum melongena L.) (Shahbaz et al., 2013). The main processes involved in this response are the osmotic adjustment (Hmidi et al., 2018; Khanna-Chopra et al., 2019) and ROS neutralization (Gao et al., 2023; Rejeb et al., 2014).
Chlorophyll reduction in salinity stress (fig. 2) can be explained by the presence of a toxic level of ions, leading to dehydration of mesophyll cells, inhibition of many enzymes involved in carbohydrate metabolism and some steps in chlorophyll synthesis (Ashraf and Harris, 2013; Tang et al., 2015). Since chlorophyll is the most important pigment for capturing light energy, its reduction will lead to a decreased photosynthesis. Therefore, measurement of chlorophyll content can be used as a plant stress indicator (Kalaji et al., 2016). The negative effect of salinity on chlorophyll content has been previously reported in melon (Sarabi et al., 2017; Yarsi et al., 2017).
mM proline treatment (fig. 2). This agrees with Kaya et al. (2007), who sprayed 10 mM proline to melon seedlings growing under salt stress. In maize plants (Zea mays L.) cultivated under salt stress, Alam et al. (2016) measured a lower reduction in chlorophyll content in plants sprayed with proline. This effect of exogenous proline was also reported in rice (Oryza sativa L.) (Bhusan et al., 2016) and was explained by an increase in antioxidant enzyme activity that prevented chlorophyll degradation. There was no increase in chlorophyll content in the 20 mM treatment. This may be due to a toxicity effect of the higher concentration that affects synthesis and accelerates degradation of this pigment (El Moukhtari et al., 2020; Verbruggen and Hermans, 2008).
CONCLUSIONS
In this study, a positive relationship was observed between the proline application and the growth of melon seedlings under salt stress. We conclude that with exogenous proline at 10 and 20 mM concentrations, plants can achieve an earlier and higher salt tolerance response. The application of this amino acid could result in an interesting strategy to increase growth under salt stress conditions, frequent in irrigated crops.