Introduction
The genus Phytophthora includes more than 100 species among the most destructive plant pathogens worldwide, causing significant economic losses in agricultura (12,17,23,31). P. palmivora is a relevant species with a wide host range including pineapple, papaya, oranges, tomatoes, tobacco, citrus, and olive trees (23). Since the early 1990s, in the main olive growing area of Argentina, several plants in different orchards were observed with initial drying of some branches followed by tree death. P. palmivora, P. nicotianae and P. citrophthora were collected from soil and roots of those symptomatic olive tree orchards (17). After a few years, the disease reached an important regional dissemination, with significant losses.
Current P. palmivora control is still based on synthetic products such as metalaxyl, fosetyl-Al, and copper-based fungicides (21,28,36). Nevertheless, these pesticides are associated with several negative consequences for the environment and human health (6,10). Furthermore, the extensive application of synthetic products, such as metalaxyl, has led to the emergence of Phytophthora-resistant strains, resulting in severe destructive disease in several crops (2,25). Considering the aforementioned, the search for greener and safer approaches represents an urgent need as well as a great challenge.
In recent decades, novel management strategies have seriously committed to environmental and human health protection. In this context, plant extracts have emerged as an interesting and promising alternative for crop protection. Plant extracts from Phlomis purpurea, Cosmos caudatus, Rosmarinus officinalis, Lavandula angustifolia, and Salvia officinalis have demonstrated an outstanding ability to inhibit Phytophthora micelial growth, sporangium, and zoospores production (25,29,33,36). Several plant bioactive compounds, including polyphenols, flavonoids, quinones, tannins, alkaloids, saponins and sterols have shown antimicrobial activity against phytopathogens (7,26).
Larrea divaricata Cav. is an autochthonous dominant shrub in South America, broadly growing in Argentina. In folk medicine, extracts of L. divaricata have been widely used as anti-inflammatory, antirheumatic, dysphoretic, amenagogic, and antimicrobial compounds (4). Even though these extracts presented antimicrobial effects against Phytophthora species, the fungicidal activity, or its efficacy as a potential protective or curative fungicide, has not yet been reported. Phytochemical reports of our group have demonstrated the presence of the same phenolic compounds with antimicrobial activity previously found in L. divaricata extracts, such as quercetin, luteolin, and cinnamic acid in L. divaricata extracts (3). However, due to the complex nature of plant extracts, attributing antimicrobial activity to a single, specific bioactive compound becomes difficult. Along with phenolic compounds, alkaloids are another relevant group of active substances present in plants.
This work studied the inhibitory activity of L. divaricata extract against P. palmivora mycelial growth, sporangia, and zoospore production. Alkaloid antimicrobial activity in the extract was analyzed against P. palmivora mycelial growth. In addition, fungicidal activity of L. divaricata extract against P. palmivora was evaluated in olive trees under greenhouse conditions.
Material and Methods
Materials, chemicals, and standards solutions
V8 agar (V8A) was prepared using 200 mL commercial V8 juice; 2 g CaCO3; 17 g agar, and 800 mL distilled water. The salt solution of Chen and Zentmyer was prepared by mixing Ca (NO3)2 (1.64g) (0.01 M); KNO3 (0.05g) (0.005 M); MgSO4 (0.48 g) (0.004 M); deionized H2O to 1 liter, and a solution of chelated iron (1 mL) consisting of (g L-1) ethylenediaminetetraacetic acid (EDTA) (13.05 g); KOH (7.5 g); FeSO4 (24.9 g) (0.16 M); and deionized water to 1 liter (2).
Analytical standards, piperine, caffeine, harmaline, nicotine, theophylline, and theobromine were purchased from Sigma Aldrich (St. Louis, MO, USA). Formic acid (85%) (FA) was obtained from Sintorgan (Bs. As., Argentina). Methanol (MeOH) and acetonitrile (ACN) of the chromatographic grade were purchased from Baker (USA). Stock solutions were prepared by dissolving each alkaloid standard at a concentration of 100 mg L-1 in methanol at 0.1% with FA. Standard working solutions of each alkaloid at concentrations of 50, 25, 10, and 1 mg L-1 were obtained from stock solutions. All these solutions were stored in dark-glass bottles at 4°C.
Plant extract
L. divaricata plants, taxonomically identified by Cátedra de Botánica de la Facultad de Ciencias Agrarias de la Universidad Nacional de Cuyo (Mendoza, Argentina), were grown in a greenhouse. Leaves were collected in the flowering period, and immediately frozen and lyophilized.
L. divaricata extract was prepared using the methodology proposed by Boiteux and collaborators (4). Bioactive compounds from L. divaricata leaves (20 g) were extracted by decoction with distilled water (200 mL) in an autoclave for 45 min at 121°C. Then the extract was filtered and the volume reduced by boiling at 20 mL. Finally, the extract was centrifuged and stored at 4°C.
Pathogen
The P. palmivora isolate was obtained from rotted rootlets of olive trees from comercial plantations in the province of Catamarca, Argentina (15), and identified by morphological and molecular characterization (17). This isolate was maintained on clarified V8 agar.
Inhibitory activity of L. divaricata extracts
The fungistatic or fungicidal activity of L. divaricata extract against P. palmivora was evaluated according to the method proposed by Mahmoudi (2017). Agar discs (4 mm in diameter) colonized by the pathogen P. palmivora (grown on V8 agar, 24°C, 5 days old) were placed at the center of Petri dishes containing V8A with different concentrations of L. divaricata extract (0; 50; 100; 150; 300; 500 and 700 mg mL-1). A positive control using a commercial fungicide metalaxyl+mancozeb (RIDOMIL GOLD 68 WG, 4 g de metalaxyl + 64 g de mancozeb, Syngenta) was included at the recommended concentration (metalaxyl 0.25 mg mL-1 for Phytophthora species). The Petri dishes were kept at 25±2°C for 4 days. After the incubation period, discs of P. palmivora from treatments showing total inhibition were transferred to plates containing V8A and incubated for 4 days at 25±2°C. Fungitoxicity of each extract concentration was assessed against mycelial growth. Thus, the plates showing P. palmivora growth indicated a fungistatic effect, while absent growth denoted fungicidal action of the L. divaricata extract. Each treatment was performed in triplicate and the test was repeated three times.
Effect of L. divaricata extract on P. palmivora sporangia and zoospores production
The effect of L. divaricata extract on P. palmivora sporulation was evaluated in vitro. Petri dishes containing 6 mL of Chen and Zentmyer solutions (11) were amended with L. divaricata extract to a final concentration of 0; 1; 5; 10; 25; 50; 100; 150; 300; 500, and 700 mg mL-1. Subsequently, three agar discs colonized with P. palmivora mycelia (4 mm in diameter, 5 days old) were placed in the extract solutions. A positive chemical control, commercial fungicide metalaxyl+mancozeb was included at the concentration described in the previous section. Each treatment was performed in triplicate and the assay was repeated three times. Petri dishes were incubated at 25 ± 2°C for 4 days under fluorescent cool-white light for sporangia induction. Agar discs were stained and fixed with acid fuchsin in 85% lactic acid. The number of sporangia per agar disk was counted using an optical microscope (200X). Results were expressed as inhibition percentage of sporangia production using equation 1.
where:
C and T = P. palmivora sporangia number of control (without treatment) and treatments, respectively
The effect of L. divaricata extract on P. palmivora zoospore production was determined after the incubation period, when the sporangium was induced to release zoospores by chilling at 4°C for 20 min, then rewarming at 25°C for 20 min. A drop of the suspensión (5 μL) was placed on a glass slide, and zoospores were counted using an optical microscope (200X). Each treatment was performed in triplicate and the assay was repeated three times. Then, inhibition percentage of zoospore production of P. palmivora was determined as follows 2:
where:
C and T = the number of P. palmivora zoospores for control (without treatment) and treatments, respectively
Determination of alkaloids from L. divaricata extract
Alkaloids (theophylline, theobromine, caffeine, piperine, nicotine, and harmaline) were determined using a HPLC-MWD system (Dionex Ultimate 3000 Softron GmbH, Thermo Fisher Scientific Inc., Germering, Germany). The detector was a Dionex MWD-3000 (RS) model. To process the obtained data Chromeleon 7.1 software was used. HPLC separations were carried out in Zorbax SB-Aq column (4.6 mm × 150 mm, 5 μm) Agilent Technologies. Ultrapure water with 0.1% FA (A) and ACN (B) were used as mobile phases. Alkaloids were separated using the following gradient: 02.7 min, 5% B; 2.710.7 min, 30% B; 10.7-11 min, 35% B; 1115 min, 50% B; 1515.5 min, 50% B: 15.516 min 30% B; 16-16.5 min 5% B; 16.517 min 5% B. The mobile phase flow was 1 mL min-1. The column temperature was held at 20°C and the sample injection volume was 5 μL. Working wavelengths for the different analytes were 254 nm, 280 nm, 320 nm, and 370 nm. Alkaloids in L. divaricata extract were identified and quantified after the comparison of retention times (tR) and absorbance values of detected peaks in the solvent with those obtained by injection of pure standards of each analyte. Alkaloid concentration was expressed as mg L-1.
Analytical quality parameters were used to assess procedure performance with the selected conditions. The linearity of the calibration curve was tested by plotting the área of reference compounds at six concentrations between 0.5 mg L-1 and 100 mg L-1 using a least-squares linear regression model. For all target compounds, correlation coefficients of calibration equations were > 0.999.
The limit of detection (LOD) and limit of quantitation (LOQ) were calculated following the International Union for Pure and Applied Chemistry (IUPAC).
Antimicrobial activity of individual alkaloids
The inhibitory effect of individual alkaloids identified by the HPLC-MWD system in L. divaricata extract was evaluated against P. palmivora using the solid agar bioassay. Thus, a 4 mm disc of P. palmivora was placed in the center of Petri dishes containing V8A amended with the alkaloids at the concentration found in L. divaricata extract. Control plates were performed simultaneously, in the growth medium without alkaloid. Petri dishes were incubated at 25±2°C for 4 days. Each treatment was performed in triplicate and the assay was repeated three times. After incubation, the P. palmivora colony area was measured using AxioVision 4.8 software. Results were expressed as inhibition percentage of mycelial growth using equation (3)
where:
C and T = the P. palmivora colony area (cm2) of control and treatments, respectively
Disease control in the bioassay
In vivo effect of L. divaricata extract was evaluated with one-year-old olive plants (Olea europaea L., var. Arauco) obtained from a commercial nursery. The assay was performed following the methodology proposed by Vettraino et al. (2009). P. palmivora inocula was prepared by mixing 20 mL millet seeds with 150 mL V8 broth in 250 mL flasks. Then, the flasks were sterilized twice and inoculated with P. palmivora (10 mycelial discs of 8 mm diameter, grown on V8 agar per flask). The flasks were incubated for 1 month in the dark at 25°C. After incubation, millet seed inoculum (20 g L-1) was added to the soil of olive plants. Control treatments only contained sterile millet seeds with V8 broth (20 g L-1). To stimulate zoospore release and disease development, pots were flooded for 48 h, then water excess was drained off. Afterwards, L. divaricata extract was added to one treatment, leaving control with only the extract. Experimental treatments were as follows: (A) inoculated soil with P. palmivora and (B) inoculated soil with P. palmivora plus L. divaricata extract at 50 mg mL-1. Controls were performed as pathogen uninoculated soil plus L. divaricata extract (50 mg mL-1) (C), and only soil (D). For each treatment, five replicates were performed and the whole assay was repeated twice. The occurrence of symptoms was observed weekly during the six months, and percentage of dead plants was calculated at the end of the experiment. After the assay, P. palmivora was reisolated from roots on a selective V8 medium (15).
Results
Inhibitory activity of L. divaricata extracts
Interestingly, all studied concentrations (50; 100; 150; 300; 500 and 700 mg mL-1) reduced mycelial growth of P. palmivora. Figure 1 (page 102), shows 50 and 100 mg mL-1 extracts had a fungistatic effect, whereas higher concentrations showed fungicidal activity.
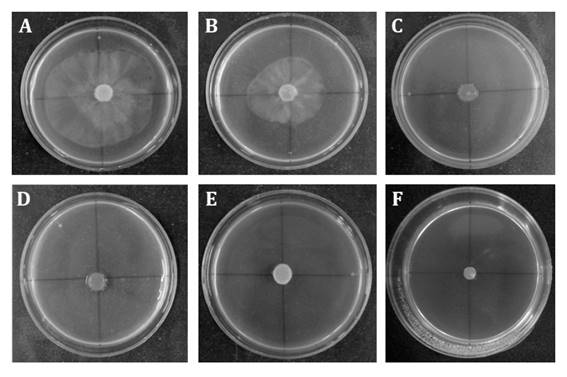
Figure 1 Fungistatic and fungicidal activity of L. divaricata extracts at different concentrations (A: control; B:50; C:100; D:150; E:300; F:500 and G:700 mg mL-1) on P. palmivora mycelial growth. Figura 1. Efecto fungicida y fungistático del extracto de L. divaricata a diferentes concentraciones (A: control; B:50; C:100; D:150; E:300; F:500 and G:700 mg mL-1) sobre el crecimiento miceliar de P. palmivora
Effect of L. divaricata extract on P. palmivora sporangia and zoospores production
Sporangia and zoospore production of P. palmivora were inhibited by L. divaricata extract (1; 5; 10; 25; 50; 100; 150; 300; 500 and 700 mg mL-1). Inhibition was dose-dependent. Figure 2 shows all bioextract concentrations were able to inhibit P. palmivora number of sporangia and zoospores to varying degrees.
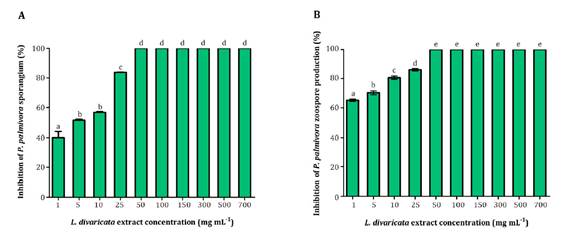
Different letters indicate significant statistical differences according to Tukey test, α < 0.05.
Letras distintas indican diferencias significativas para tets de Tukey, α < 0,05
Figure 2 Effect of L. divaricata extract on P. palmivora sporangia (A), and zoospore production (B) at different concentrations. Figura 2. Efecto de diferentes concentraciones del extracto de L. divaricata sobre la producción de zoosporangios (A) y zoosporas (B) de P. palmivora
Interestingly, at the lowest concentration evaluated (1 mg mL-1), the extract inhibited 40% and 60% of sporangia and zoospore production, respectively. Noteworthy is that 50 mg mL-1 of the extract completely suppressed sporangia and zoospore production.
Determination of alkaloids from L. divaricata extract
Piperine, caffeine, harmaline, nicotine, theophylline and theobromine in L. divaricata extract were determined by chromatographic method HPLC MWD. Table 1 shows analytical quality parameters. All of them showed a linear range from the LOQ up to at least 100 mg L-1.
Table 1 Analytical figure of merit for alkaloids analysis by HPLC-MWD method. Tabla 1. Cifras de mérito analítico para el análisis de alcaloides mediante HPLC-MWD
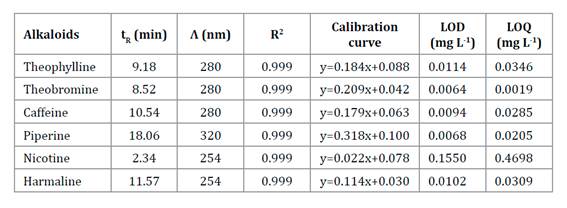
tR: retention time; Λ: wavelength; R2: coefficient of determination; LOD: limit of detection; LOQ: limit of quantitation.
tR: tiempo de retención; Λ: longitud de onda; R2: coeficiente de determinación; LOD: límite de detección; LOQ: límite de cuantificación.
The only alkaloid detected was piperine; the other five analytes were not detected, considering the analytical methodology used. Piperine concentration was 44.02 mg mL-1.
Alkaloid antimicrobial activity by solid agar bioassay
Since the natural concentration of piperine in L. divaricata extract at 50 mg mL-1 was 2.20 mg mL-1, the biological activity of this alkaloid was determined at 0.04, 2.20 and 22.01 mg mL-1. As shown in Figure 3, the highest inhibition observed for piperine was 75% at 22.01 mg mL-1.
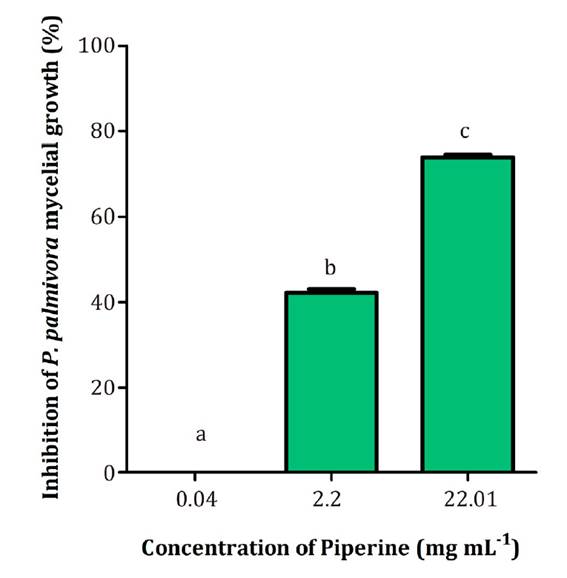
Different letters indicate significant statistical differences according to the Tukey test, α < 0.05.
Letras distintas indican diferencias significativas para test de Tukey, α < 0,05.
Figure 3 Effect of piperine on P. palmivora mycelial growth at different concentrations. Figura 3. Efecto de diferentes concentraciones de piperina sobre el crecimiento miceliar de P. palmivora
Interestingly, a 10-fold lower piperine concentration (2.20 mg mL-1) achieved 42% inhibition.
Disease control in the greenhouse
Inoculated soil with P. palmivora showed 73% dead plants (treatment A). When the soil was inoculated with P. palmivora plus L. divaricata extract at 50 mg mL-1, total dead plants percentage was only 27% (treatment B) (Figure 4).
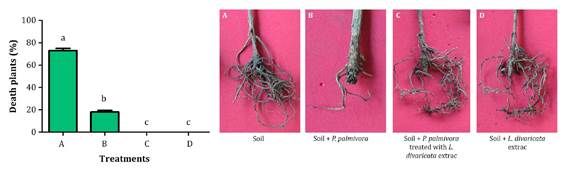
Different letters indicate significant statistical differences according to the Tukey test, α < 0.05.
Letras distintas indican diferencias significativas entre los tratamientos según test de Tukey, α < 0,05.
Figure 4 Percentage of dead olive plants after treatments at 120 days (A: soil infected with P. palmivora; B: soil infected with P. palmivora plus L. divaricata extract at 50 mg mL-1; C: soil with L. divaricata extract; D: only soil. Figura 4. Porcentaje de plantas de olivo muertas después de 120 días del tratamiento (A: suelo inoculado con P. palmivora; B: suelo inoculado con P. palmivora y tratado con extracto de L. divaricata a una concentración de 50 mg mL-1; C: suelo tratado con extracto de L. divaricta; D: suelo solo sin tratar ni inocular
These results highlight the control potential of L. divaricata extract. Control efficacy of the extract against P. palmivora was 63% (1). Remarkably, no significant differences were observed between merelysoil (D) and soil containing only the extract of L. divaricata (C). In all cases, P. palmivora was re-isolated from the inoculated plants.
Discussion
The current study evaluated the efficacy of L. divaricata extract for control of P. palmivora in vitro and in olive trees under greenhouse conditions. Plant extracts, their bioactive compounds and the ability to inhibit pathogens or provide medicinal properties have always been of great interest to researchers. L. divaricata extracts are a rich source of bioactive compounds against a wide range of microorganisms (1). In this sense, there is scarce information on the chemical composition and antimicrobial activity of individual compounds against Phytophthora species. A previous study by our group reported that four plant extracts showed antimicrobial activity against P. palmivora mycelial growth, with L. divaricata extract being the most effective (3). However, to the best of our knowledge, the fungicidal or fungistatic effect of L. divaricata extract against P. palmivora mycelial growth has not been reported yet. In this work, the extract showed fungistatic effect at a concentration between 50 and 100 mg mL-1, and fungicidal effect between 150 and 700 mg mL-1.
Different studies have reported the fungicidal activity of L. divaricata extracts against different pathogen species. Vogt et al. (2013) studied the efficacy of L. divaricata organic extracts against Fusarium graminearum, F. solani, F. verticillioides and Macrophomina phaseolina. F. graminearum and M. phaseolina were completely inhibited at 0.25 mg mL-1 of L. divaricata chloroform extract (33). Hapon et al. (2017) demonstrated that 200 mg mL-1 of L. divaricata extract inhibited mycelial growth of Botrytis cinerea (94%) (13). Interestingly, in this work, a considerably lower concentration of L. divaricata extract was able to completely inhibit P. palmivora growth.
The asexual stages, including sporangia and zoospore, are the main propagules involved in the rapid spread of P. palmivora. Therefore, evaluating the efficacy of extracts in controlling these asexual structures is of utmost importance. Particularly, in our study, L. divaricata extract showed inhibitory activity on both sporangia formation and zoospore production of P. palmivora. Salehan et al. (2013) reported that Cosmos caudatus aqueous extract at 200 mg mL-1 inhibited 37% sporangia formation and 23% zoospore production of P. palmivora (29). Widmer and Laurent (2006) demonstrated that plant extracts of Rosmarinus officinalis, Lavandula angustifolia and Salvia officinalis at 250 mg mL-1 were able to inhibit zoospore production of P. capsici, P. megakarya and P. palmivora (35). In line with these studies, our results also highlight the efficacy of L. divaricata extract in inhibiting sporangia and zoospore production of P. palmivora.
In view of the above, L. divaricata extract represents a great alternative for the in vitro control of P. palmivora. Noteworthy is that the antimicrobial activity of L. divaricata extract has been related with the presence of phenolic compounds (3). In this study, piperine was the only alkaloid found in the extract by HPLC-MWD analysis. This alkaloid is a bioactive component with outstanding biological properties such as antitumoral, anti-inflammatory, antifungal and antimicrobial (14,20,30).
Several studies have documented the antibacterial activity of piperine against Escherichia coli, Klebsiella pneumonia, Salmonella enterica, Staphylococcus aureus, S. epidermidis, Enterococcus faecalis and Bacillus subtilis (9,16,22,27,37). Marques et al. (2010) reported antifungal activity of this compound against Cladosporium cladosporioides and C. sphaerospermum (19). Moon et al. (2016) demonstrated that piperine inhibited mycelial growth and aflatoxin production of Aspergillus flavus (24). Wang et al. (2020) demonstrated the fungicidal activity of piperine against Rhizoctonia solani, Fusarium graminearum, Phomopsis adianticola, Alternaria tenuis, Phytophthora capsici and Gloeosporium theaesinensis. Piperine at 0.1 mg mL-1 inhibited mycelial growth of P. capsici at 42% (35). These results agree with those presented in this work, where piperine at 2.20 mg mL-1 inhibited P. palmivora mycelial growth by 42 %. To the best of our knowledge, this constitutes the first report on antimicrobial activity of piperine against P. palmivora. Interestingly, we found that the inhibitory activity of L. divaricata extract against P. palmivora was higher than piperine used at the same concentration. This results indicate that piperine could partially explain extrat total bioactivity against this pathogen. Unfortunately, no studies have used piperine in the concentration range tested in our study against P. palmivora. Considering that plant extracts are complex matrices, it is logical to assume that different compounds of L. divaricata secondary metabolism, could be active against several plant pathogens.
As seen in our in vitro studies, the extract was also effective when the biological activity was assessed on healthy olive plants artificially inoculated with P. palmivora under greenhouse conditions. In the latter case, the number of dead plants was significantly reduced compared to non-inoculated plants. Previous studies have also demonstrated the efficacy of plant extracts in controlling soilborne pathogens (5,34). Bowers et al. (2004) showed that synthetic cinnamon oil, pepper and cassia extracts reduced P. nicotianae populations on infected Catharanthus roseus (L.) plants under greenhouse conditions (5). Wang et al. (2019) demonstrated that zedoary turmeric oil has excellent antifungal activity against P. capsica, inoculated on detached cucumber leaves (34). These results are consistent with those presented in this work, where L. divaricata extract showed control activity against P. palmivora in olive plants.
Conclusion
This work highlights the potential of L. divaricata extract for sustainable management of P. palmivora. The extract obtained had an effective inhibitory activity on mycelial growth, sporangia and zoospore production of this pathogen. We also demonstrated that the presence of the alkaloid piperine in the extract, together with other phenolic compounds, contributes to antimicrobial activity. These results support the use of L. divaricata extract as another environmentally friendly tool for an integrated disease management programme against dry branch disease caused by P. palmivora. Further complementary studies are required to evaluate commercial applications and effects on beneficial soil organisms.