KEY POINTS
• Chia and flax integral flours are a rich in ALA.
• Supplemented diets contribute to differen tial ω-3 PUFAs profile in tissues.
• The dietary intake of integral flours ame liorated systemic inflammation.
Omega-3 polyunsaturated fatty acids (ω-3- PUFAs), in addition to essential nutrients, have been associated with potential health benefits1-2. There are two types of ω-3- PUFAs, known as short-chain ω-3 PUFAs like ALA (alpha-linolenic acid, C18:3 ω-3) or long-chain ω-3 PUFAs such as EPA (eicosapentaenoic acid, C20:5 ω-3) and DHA (docosahexaenoic acid, C22:6 ω-3). Short-chain ω-3 PUFAs are present in plant oil such as flax and chia seeds, while long-chain ω-3 PUFAs are usually found in marine products1-2. Although short-chain ω-3 PUFAs are more common and less expensive, the potential health benefits of ω-3 PUFAs have been related only to long-chain ω-3 PUFAs1. Compelling data from epidemiologi cal and interventional studies have demonstrat ed an inverse correlation between long-chain ω-3 PUFAs and the risk of suffering from some chronic inflammatory diseases1.
In the Western diet, the most consumed ω-3 PUFA is ALA. It is considered an essential fatty acid because it can not be synthesized by humans1. It bioconverts in the liver to EPA and DHA and to a lesser extent in the brain to DHA, a process that involves several steps orchestrated by multiple elongases, desatu rases, and β-oxidases2-5. The efficient conver sion of short to long-chain ω-3 PUFAs (EPA and DHA) in the liver can increase the levels of these fatty acids in critical tissues such as the brain, cardiac, and skeletal muscles, where they exert their protective effects and provide health benefits1,6,7.
Omega-3 fatty acids are well-known dietary supplements that optimize physiological func tions and alleviate pathological conditions, in cluding inflammation5. Moreover, long-chain ω-3 fatty acids (EPA and DHA) have been shown to promote an anti-inflammatory eicosanoid profile and NF-kB gene expression, thereby re ducing pro-inflammatory cytokines8-11. In this study, a murine model of systemic inflamma tion induced by lipopolysaccharide (LPS) was implemented12-14.
Chia and flax seeds have been studied in vari ous research models for their potential to allevi ate systemic inflammation in human diseases4,5,15,16. Previous dietary studies often utilized whole seeds, defatted flours, or seed oils4,15,17. However, after an extensive literature review, we did not find any studies reporting the dietary supplemen tation of integral chia and flax flours and their ef fects on EPA and DHA levels in crucial tissues, as well as their potential role as anti-inflammatory agents.
This work aimed to evaluate the anti-inflam matory effect of dietary supplementation with integral chia and flax flours rich in ALA in the amelioration of LPS-induced systemic inflammation in a murine model.
The hypothesis is that the dietary intake of integral chia and flax flours would contribute to attenuating inflammation through various mechanisms evaluated by multiparametric assays.
Material and methods
Animals
Male Balb/c mice (25-30 g, n: 30, age: 3 weeks) were ob tained from the animal house of the Facultad de Medici na, Universidad Nacional del Nordeste (UNNE), Argentina. All animals were housed under standard laboratory con ditions with a room temperature of 25.0 ± 2.0 °C, relative humidity of 55-65%, and a 12 h light/dark cycle. They had ad libitum access to food and water. In vivo experiments were conducted in accordance to the Institutional Ani mal Care and Use Committee (IACUC) of the Facultad de Medicina, UNNE (Resolution #0002-IACUC/17), followed the ARRIVE guidelines (Animal Research: Reporting of in vivo Experiments) and the National Institutes of Health guide for the care and use of laboratory animals (NIH Pub lications No. 8023, revised 1978).
Experimental procedures
The experimental setup included three groups with 10 mice per group: (I) control group on diet A (balanced commercial murine chow, Cooperación S.A.®, Buenos Ai res, Argentina), (II) diet B (supplemented with integral chia flour), and (III) diet C (supplemented with integral flax flour). Experimental diets B and C were formulated to provide the recommended ω-3 contribution for murine nutrition18. The mice were provided with food ad libitum for 70 days (10 weeks).
Samples sizes were based on prospective power anal ysis (80%), using the magnitude of group differences and the standard errors within each group observed in our preliminary studies.
Initially, the macronutrients centesimal composition of the integral flours and the experimental diets were de termined (Table 1).
The recommended intake of ω-3 fatty acids (FA) for mice is about 1.3 g ω-3 FA/kg diet19. The experimental di ets B and C were supplemented by doubling the recom mended amounts of ω-3 FA (2.6 g /kg diet). The nutritional composition of diets B and C is presented in Table 2.
The FA profiles of the integral flours (Table 3) and the diets (Table 4) were determined using gas chromatography and mass spectrometry (GC/MS). The FA methyl esters (FA MEs) were identified by comparing their retention times to those of commercial standards and those provided by the International CYTED Net (208RT0343) GC/MS.
The body weight and diet consumption of mice were measured weekly using an Ohaus portable balance (TA 3001) with a precision of 0.1g.
At the end of the experimental period, five animals from each group were injected with LPS (serotype 0127: B8, Sigma, St. Louis, MO, USA) at a dose of 1.3 mg/kg i.p20 to induce systemic inflammatory condition. After 24 h, the mice were anesthetized with pentobarbital (60 mg/kg i.p) to obtain blood samples by cardiac puncture. Eutha nasia was performed by cervical dislocation. These mice were used to obtain different tissue samples for further assays.
The remaining five unstimulated mice were injected with the same volume of sterile saline solution and used to obtain peritoneal macrophages for primary cultures stimulated with LPS (section 2.5).
Hematologic parameters and biochemical determinations
Biochemical determinations were carried out using a spectrophotometer (Metrolab 1600 Plus) and Wiener Lab reagents. The measurements included total proteins, al bumin, triglycerides, total cholesterol, and glucose mea surements in plasma samples. Hematological parameters were evaluated using a Mendray BC 2800 VeT adjusted to murine standard values.
Phagocytic capacity assay of peritoneal murine macrophages (Mø)
A non-invasive assay using colloidal carbon parti cles was performed to evaluate the phagocytic capacity through the morphological changes of cultured adherent cells21. The peritoneal macrophages of mice previously stimulated in vivo with LPS were used.
Briefly, the outer layer of the peritoneum was care fully cut and the peritoneal cavity was gently exposed. The peritoneal lavage was performed with 5 ml of ster ile phosphate buffer saline pH 7.2 (PBS). Cell pellets were washed and Mø were placed over sterile degreased glasses in 35 mm culture dishes. Then, 30 μl of Pelikan #17 Schwarz Black colloidal carbon particles (ref. 70306 4340503), were added to each dish. The medium was re moved after 60 min of incubation at 37°C. Adherent cells were washed twice with 1 ml of phosphate-buffered sa line (PBS) for removing the excedent of medium and col loidal carbon particles.
The cells were fixed with a 0.4% paraformaldehyde solution. A semi-quantitative evaluation was obtained by the measurement of the % intracellular colloidal carbon area, determined by the ImageJ 1.53s software (Wayne Rasband and contributors. National Institutes of Health. USA). Images were taken using an Optika Italy-B-290 mi croscope with a built-in camera. Five random fields per sample were processed (X400 original magnification).
Obtention of murine peritoneal macrophages (Mø) and setting of primary cell cultures
Peritoneal macrophages without in vivo challenge of LPS were collected as described in 2.4. Cells were seeded in 24-well plates with Dulbecco’s modified Eagle’s medi um (DMEM) containing 10% fetal bovine serum and 1% penicillin/ streptomycin. Cells were cultured for 2 h at 37°C in a 5% CO2 humidified atmosphere. Mø enrichment (≥ 95%) was confirmed by nonspecific esterase staining and viability (≥ 95%) was determined by the trypan blue exclusion assay22. Subsequently, LPS at a concentration of 1 μg/mL (Lipopolysaccharide Escherichia coli O127:B8, γ-irradiated, Sigma, Darmstadt, Germany) was added to the cell suspension and incubated for 24 h to achieve complete activation23.
NF-κB immunodetection
The detection of the nuclear translocation of phos phorylated NF-κB p65, serving as a biomarker of systemic inflammation, was performed using immunocytochem istry (ICC) in samples of murine primary macrophages stimulated in vitro with LPS. Cells were fixed with anhy drous methanol (99.8%) for 1 min. Subsequently, hydra tion was carried out using a series of decreasing alcohol concetrations.
Liver and kidney samples of mice fed experimental di ets were assessed for NF-kB localization by immunohis tochemistry (IHC). The samples were fixed with buffered formalin at room temperature. After fixation, the samples were dehydrated using increasing concentrations of al cohol and embedded in paraffin. Sections (3 μm) were deparaffinized and rehydrated. To inhibit endogenous peroxidase activity and prevent not specific labeling, the samples for ICC and IHC were incubated with 0.3% H2O2 in methanol for 10 min, followed by treatment with a blocking solution for 30 min.
The samples were then incubated with an NF-κB p65 antibody (A: sc-109 Santa Cruz Biotechnology CA, USA, dilution 1: 250) for 18 h at 4 °C. Immunostaining was per formed using a DAKO LSAB+/HRP kit (Dako Cytomation) followed by the application of a chromogen DAB (DAKO kit) according to the manufacturer´s instructions. Images were captured using an Optika Italy-B-290 microscope with a built-in camera. The percentage of immunoreac tive area for NF-κB p65 (μm2) was measured in five ran dom fields (x1000 original magnification) using the Image J software (National Institutes of Health, Bethesda, MD)24.
Tissular Omega-3 profile
The ω-3 FA profile was analyzed by GC-MS to deter mine ALA, EPA, and DHA levels in cardiac muscle, liver, skeletal muscle, and brain.
The FA composition of the tissues was determined by gas chromatography using a Shimadzu (GC 2014) chro matograph equipped with a flame ionization detector. Analyses were carried out with a capillary column CP Sil 88 (100 m, 0.25 μm film thickness)25. The carrier gas was hydrogen with a split ratio of 1:10. The column tem perature was held at 75°C for 2 min after injection, then 5°C/min to 170°C, held for 40 min, 5°C/min to 220°C and held for 40 min. The injection volume was 0.5 μL, and the column flow was 0.8 mL/min. The fatty acid methyl esters (FAMEs) were formed by transesterification with methanolic potassium hydroxide solution as an interim stage before saponification (ISO 5509:2000, Point 5 IUPAC method 2.301).
Briefly, samples from different tissues were excised af ter euthanasia, washed extensively with cold saline solu tion (0.9% NaCl), and homogenized mechanically with a polytron tissue homogenizer before total lipid extraction according to Bligh & Dyer26. The internal standards were added before lipid extraction. The detection limit for the main FAMEs identified ranged from 0.01% to 0.03%25.
FAMEs were identified by comparison of their reten tion times relative to those of commercial standards. The values of FA content were expressed as the percentage of total FAMEs.
Real-time polymerase chain reaction analysis
Hepatic, renal, and splenic samples were preserved in RNA later (Sigma, St. Louis, MO, USA). Total RNA was extracted using Transzol reagent (TransGen Biotech Co., Beijing, China) according to the manufacturer’s instruc tions. The reverse transcription of RNA was performed using M-MLV transcriptase (Promega, Madison, WI, USA) following technical guidelines. Gene expression was quantified in ECO® Real-Time PCR System (Illumi na, San Diego, CA, USA) using HOT FIREPol®EvaGreen® qPCR mix (Solis Byodine, Tartu, Estonia) according to the product’s datasheet. The primers were as follows (5′-3′): IL-1β-F, GAGCCCATCCTCTGTGACTC; IL-1β-R, TC CATTGAGGTGGAGAGCTT; IL-6-F, ATGAAGTTCCTCTCTG CAAGAGACT; IL-6-R, CACTAGGTTTGCCGAGTAGATCTC; TNF-α- F, ATGAGCACAGAAAGCATGATC; TNF-α- R, TA CAGGCTTGTCACTCGAATT; IL-10-F, CAGCCGGGAAGA CAATAACT; IL-10-R, TCATTTCCGATAAGGCTTGG; GAP DH-F, TGATGACATCAAGAAGGTGGTGAAG; GAPDH-R, TCCTTGGAGGCCATGTGGGCCAT.
The relative expression of IL-1β, IL-6, TNFα, and IL-10 was normalized to that of GAPDH and calculated using the ΔΔCt method.
Statistical analysis
Data are expressed as the mean ± standard deviation (SD). Comparisons between groups were performed using ANOVA with post hoc Bonferroni´s multiple comparisons tests. The software used was GraphPad Prism 8.0 (GraphPad, San Diego, CA, USA). Differences between groups were statistically significant at p<0.05.
Results
Nutritional parameters
The increment in body weight was com parable among all experimental groups (Fig. 1A). Figure 1B illustrates a significant decrease (p < 0.0001) in food intake noticed between ex perimental groups fed diets B and C compared to the control (diet A). However, the feed conver sion index did not show statistically significant differences. Hence, the nutritional efficiency of diets B and C were similar (data not shown).
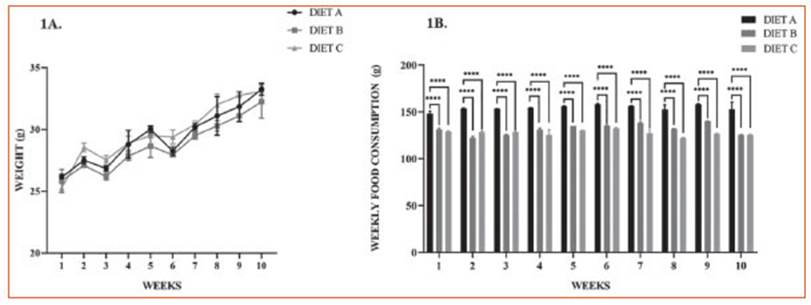
Figure 1 Nutritional parameters. Lines represent 1A. Mice body weight (g), 1B. Weekly food consumption (g) of mice fed diet B and C compared to control (diet A) for 70 days (10 weeks) of food treatment. Data are expressed as the mean ± SD from three independent experiences (n = 10 mice/group). Differences among groups were analyzed by two-way ANOVA with Bonferroni’s multiple comparisons tests
Mice showed a healthy status without any pathological manifestation through the experi mental study.
Hematological parameters and biochemical determinations
Hematological data revealed that hemato crit, hemoglobin content, total white blood cell counts, and the differential leukocyte contribu tion did not change significantly among the experimental groups (Table 5).
Animals fed diets B and C exhibited a signifi cant decrease (p < 0.05) of plasma glucose, total cholesterol, and triglycerides levels compared to diet A (control). Even though, total protein and albumin concentrations did not show signifi cant differences (Table 5).
Phagocytic capacity assay of murine peritoneal macrophages (Mø)
This assay was performed to evaluate the effect of ALA from experimental diets on the phagocytic capacity of peritoneal macro phages through the incorporation of colloidal carbon particles after LPS stimulation. The use of this inert model avoids the use of fresh Zy mosan particles or any other type of noxa that can induce additional inflammatory trigger ing27.
Figure 2 shows representative microphoto graphs of peritoneal Mø from experimental ani mals following LPS-induced systemic inflamma tion (1.3 mg/kg). Experimental data revealed that the Mø of groups B and C exhibited an increased percentage of the intracellular colloidal carbon area compared to the control.
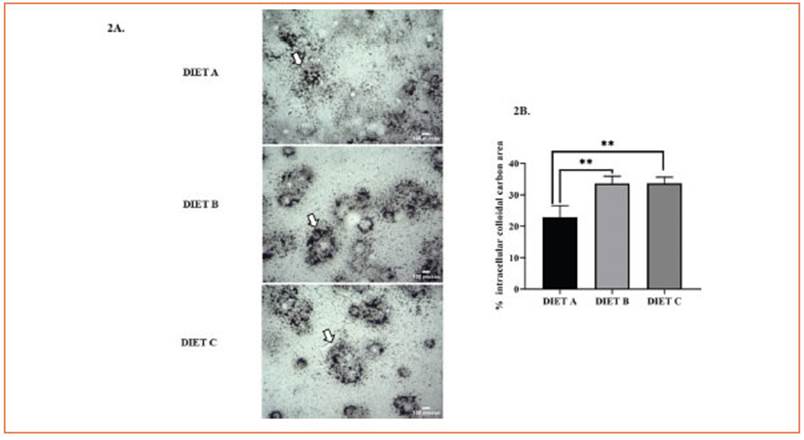
Figure 2 Phagocytic capacity assay of murine peritoneal macrophages. A: Phagocytosis assay with colloidal carbon particles. Representative images corresponding to primary macrophages from mouse intraperitoneal lavages 24 hours after in vivo stimulation with LPS: control group (diet A), animals treated with a diet based on integral chia flour (diet B), and animals treated with a diet based on integral flax flour (diet C). Magnification X400. Arrows indicate colloidal carbon particles engulfed by peritoneal macrophages. The bars represent 100 μm. B: Semi-quantitative analysis of the % intracellular colloidal carbon area. The results are expressed as the mean ±SD. Image processing to evaluate the intracellular colloidal carbon area was performed using Image J software (National Institutes of Health, Bethesda, MD). Differences among groups were analyzed by one-way ANOVA with Bonferroni’s multiple comparisons tests. (**p< 0.01)
NF-κB immunodetection
NF-κB is considered a key member of the orchestrated signaling pathway following LPS stimulation25. Once activated, free NF-κB dimers translocate to the nucleus stimulating pro-inflammatory cytokine genes transcription, as well as other target genes.
In this study, the nuclear NF-kB immunode tection significantly decreased (p < 0.0001) in peritoneal macrophages from animals fed-diet B and C compared to the control (Fig. 3A).
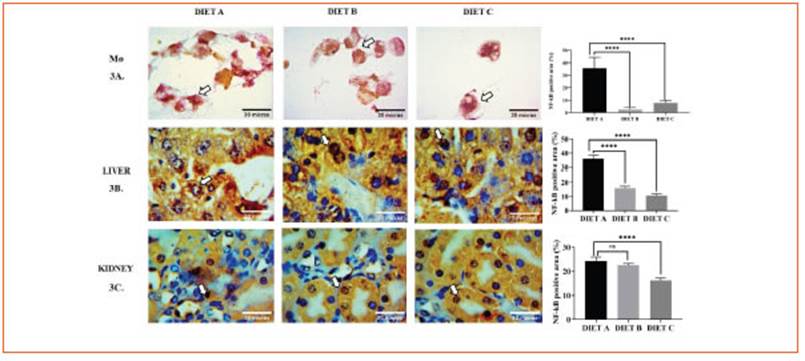
Figure 3 Immunodetection of the transcription factor NF-κB in primary macrophages, liver and kidney. A: Immunocytochemistry of NF-kB in primary macrophages 24 h after in vitro induction of systemic inflammation with LPS. Representative images correspond to the control group (diet A), animals treated with a diet based on integral chia flour (diet B), and animals treated with a diet based on integral flax flour (diet C). Arrows indicate intranuclear localization of the p65 NF-κB subunit. The immunoreaction was intense in the nuclei of Mø of group A. Magnification X1000. The bars represent 30 μm. B: Immunohistochemical assay for immunodetection of the nuclear transcription factor NF-κB in liver. C: Immunohistochemical assay for immunodetection of the nuclear transcription factor NF-κB in kidney. Representative images corresponding to the liver (3b) and kidney (3c) from mice stimulated in vivo with LPS: control group (diet A), animals treated with a diet based on integral chia flour (diet B), and animals treated with a diet based on integral flax flour (diet C). Representative photomicrographs at X1000 magnification are shown. The bars represent 30 μm. Semi-quantitative analysis of the % positive NF-kB area (μm2) was measured in five random fields for each group using the Image J software (National Institutes of Health, Bethesda, MD). The results are expressed as the mean ±SD. ANOVA and Bonferroni test (****p<0.0001)
Thus, our findings suggest that integral chia and flax flours may reduce NF-κB translocation from cy tosol to nucleous more effectively than diet B.
Figure 3 (Fig. 3B) illustrates the immunodetec tion of the transcription factor NF-κB in the liver after induction of systemic inflammation. NF-κB positive area showed a significant decrease in mice fed diets B and C (p < 0.0001) against the control, suggesting their potential anti-inflam matory effect (Fig. 3B).
Furthermore, NF-κB immunoreactivity in renal tissue exhibited a significant decrease (p<0.0001) in diet C-fed mice (Fig. 3C). No statis tical differences were found for diet B versus the control.
Tissular Omega-3 profile
The differential ω-3 FA profile was deter mined in various tissues, including cardiac (Fig. 4A), hepatic (Fig. 4B), skeletal muscle (Fig. 4C), and nervous tissue (Fig. 4D).
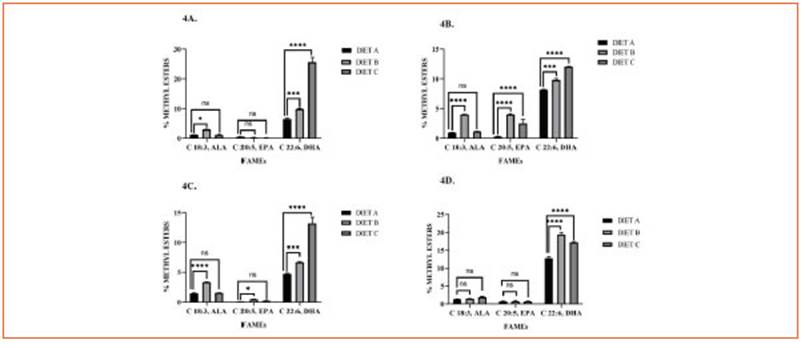
Figure 4 Tissular Omega-3 profile. Bars represent methyl esters (%) of ALA (C 18:3, ω-3), EPA (C 20:5, ω-3), and DHA (C 22:6, ω-3) in cardiac muscle (4A), liver (4B), skeletal muscle (4C), and brain (4D) of mice fed with diet A, B, and C. Data are expressed as mean ± SD from three independent experiences (n = 10 mice/group). Differences among groups were analyzed by two-way ANOVA with Bonferroni’s multiple comparisons tests (*p < 0.05; ***p < 0.001; ****p < 0.0001)
In terms of ALA levels, there were no signifi cant variations observed in all tissue samples with diet C supplementation. However, con sumption of diet B significantly increased ALA concentrations in the liver, cardiac muscle, and skeletal muscle, but not in the brain.
EPA levels showed a significant increase in he patic tissue with both diets B and C, while only diet B promoted an increase in skeletal muscle.
DHA emerged as the predominant ω-3 FA in the liver, heart, and skeletal muscle of animals fed diet C compared to diet B. However, the diet B group exhibited a significant increment of DHA levels in the brain (p < 0.0001).
Expression of IL-1β, IL-6, TNF-α and IL-10 in renal, hepatic, and splenic tissues
To evaluate the anti-inflammatory effects of integral chia and flax flours, the expression of IL-1β, IL-6, TNF-α, and IL-10 were determined in liver, kidney and spleen by RT-qPCR.
These cytokines expression decreased in he patic and renal tissue from mice fed-experimen tal diets compared to diet A, suggesting an anti-inflammatory effect of chia and flax flours rich in ALA. These findings are consistent with the reduction of NF-κB signaling pathway activa tion mentioned earlier. Additionally, in splenic tissue, IL-1β and IL-6 expression increased while TNF-α decreased (Fig. 5).
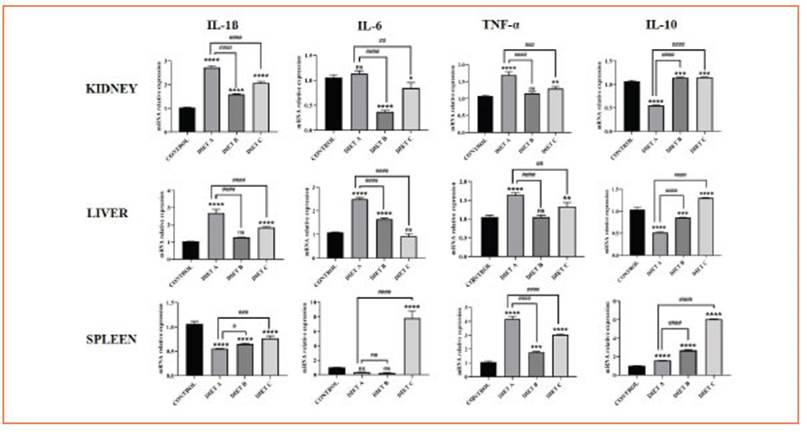
Figure 5 The effect of chia and flax flours in LPS-mediated expression of IL-1β, IL-6, TNF-α and IL-10 in the kidney, liver, and spleen. Values are presented as mean ± standard deviation (SD) of three independent experiments, corresponding to the control group (diet A), animals treated with a diet based on integral chia flour (diet B), and animals treated with a diet based on integral flax flour (diet C). Differences among groups and control were analyzed by one-way ANOVA with Bonferroni’s correction (*p<0.05; **p<0.01; ***p<0.001; ****p<0.0001). Differences among experimental groups (diet B and C) and diet A were analyzed by one-way ANOVA with Bonferroni’s correction (#p< 0.05; ##p< 0.01; ###p< 0.001; ####p< 0.0001). RT-qPCR data were calculated using the ΔΔCt method using GAPDH as a reference gene
The expression of IL-10, an anti-inflammatory cytokine, was significantly increased (p < 0.0001) in renal, hepatic, and splenic tissues, further re inforcing the anti-inflammatory role of the chia and flax-supplemented diets (Fig. 5).
Discussion
This study describes the anti-inflammatory effect of dietary supplementation with integral chia and flax flours rich in ALA on systemic in flammation induced by LPS.
The mode of incorporation of these seeds rep resents an innovative approach to studying their properties with potential application in food matrices since other reports were performed with seed oils28-31 or seed-defatted flours.
The experimental diets were designed ac cording to the animal´s requirements for Balb/c mice18-4. The nutritional parameters of our ex perimental data were close to normality for the mice strain, sex, and age as described by the NRC - The Laboratory Animals18.
On the other hand, the hematologic param eters did not change significantly and were co incident with Kelada et al32 and according to ref erence values18-33. The biochemical parameters (glucose, total cholesterol, and triglycerides) sig nificantly decreased in diets B and C compared to the control (diet A) in agreement with other studies29-34.
Extensive data demonstrate that ALA is en dogenously transformed into EPA and DHA, the main bioactive forms of ω-3 FA, with a higher conversion rate occurring in the liver than in the brain6-14.
It is well known that DHA and EPA are pres ent in varying proportions across different tis sues31,35-38 and are strongly associated with anti-inflammatory effects6-14.
However, there is limited knowledge regard ing the ω-3 FA profiles in crucial tissues of mice fed with integral chia and flax flours rich in ALA35.
The present study revealed variations in ALA, DHA, and EPA levels among crucial tissues through dietary supplementation.
Our results suggested that DHA was the pre dominant bioactive derivative of ALA in the hearts of mice fed experimental diets, mainly through flax supplementation. Furthermore, both EPA and DHA were found in hepatic tissue. These findings agree with Watkins’s work39.
On the other hand, our study revealed that DHA was the predominant fatty acid in brain tissue, which is consistent with the findings of Valenzuela et al30. Their study demonstrated that ALA is converted to DHA and EPA in the brains of mice fed diets containing chia, flax, and/or salmon oils. However, to our knowledge, there are no reports available regarding the ω-3 FA profile in murine skeletal muscles from diets including integral chia and flax flours.
Our results demonstrated that the chia diet predominantly increased levels of EPA and DHA in skeletal muscles. In contrast, the flax diet only promoted the presence of DHA. These findings align with the study conducted by Apperson and Cherian40, which showed the prevalence of DHA, AA (c20:4 ω-6), and ALA in bird’s muscles after feeding them with flax seeds.
Despite the extensive evidence regarding the anti-inflammatory role of ω-3 fatty acids, the specific effect of dietary supplementation with integral chia and flax flours has not been thor oughly investigated6-14. Therefore, experiments were conducted to examine the potential alle viation of systemic inflammation in an LPS-in duced murine model.
The experimental groups showed an en hanced phagocytic capacity of peritoneal macrophages. It has been suggested that the phagocytic plasticity of macrophages may be associated with changes in the composition and structure of the cell membrane resulting from the incorporation of ω-3 fatty acids5-41. Furthermore, Gutierrez et al5 described that the dietary intake of ω-3 fatty acids (ALA, EPA, and DHA) leads to macrophage polarization from a pro-inflammatory M1 phenotype to an anti-in flammatory M2 phenotype. Although this aspect was not specifically addressed in our study, it is well-documented that M2 macrophages exhibit higher rates of phagocytosis5,42,43.
In parallel, the NF-κB transcription factor plays a crucial role in regulating the immune re sponse by controlling the transcription of genes involved in inflammation, including cytokines/ growth factors, soluble cytokine receptors, ad hesion molecules, and immunoregulatory mol ecules31,42,44. In our study, the immunodetection of p65 NF-κB in critical tissues and peritoneal macrophages decreased in the experimental groups compared to the control group after LPS challenge. It is worth noting that although the diets contained the same amount of ALA, de rived from different food sources (integral chia flour vs integral flax flour), their potential for downregulating NF-κB immunoreactivity varied among the tissues. However, a recent study by Branco Ramos Nakandakari45 investigated the protective effects of flax oil but did not find a re duction in the expression of inflammatory me diators in the murine liver. In contrast, our work revealed that integral flax flour exerted a protec tive effect in the context of systemic inflamma tion. Further studies are necessary to identify the mechanisms involved in this finding.
The anti-inflammatory effects of the experi mental diets were also demonstrated by a signif icant decrease in the expression of IL-1β, TNF-α, and IL-6 in hepatic and renal homogenates. These results are consistent with other studies that have reported the downregulation of pro-inflammatory cytokines following dietary intake of ALA in various animal models17,18,46-48. Howev er, it is important to note that the expression of IL-6 and IL-1β increased in spleen homogenates. In this regard, the study by Svahn et al49 reported an increase in IL-16 levels in the spleen, while this pro-inflammatory cytokine decreased in cir culation. These findings suggest that the regu lation of pro-inflammatory cytokines may be more localized rather than systemic in splenic tissue. Our results reveal that the experimental diets have different capacities to downregulate the production of pro-inflammatory cytokines, highlighting the need for further research to elucidate the underlying mechanisms. The expres sion of IL-10 increased in the kidney, liver, and spleen following the dietary intake of integral chia and flax flours, which is consistent with the findings of Al Za’abi et al50. IL-10 is known to be a regulatory cytokine that inhibits inflammatory responses, thereby protecting tissues from damage.
Our results indicate that diets supplemented with integral chia and flax flours maintain the normal nutritional status of animals, improve metabolic biochemical parameters, and contrib ute to a differential ω-3 PUFA profile in critical tissues. Furthermore, the present work demon strates that these integral flours alleviate sys temic inflammation induced by LPS.
Further research is needed to establish the mechanisms involved to obtaining efficient ALA bioconversion rates in different species of mammals, including humans, for make direct associations with the improvement of differ ent pathologies or the optimization of different physiological phenomena. Additionally, it is cru cial to determine the independent rols of ALA51.
In conclusion, this study can contribute to strengthening the scientific evidence necessary for the future development of nutraceutical supplements derived from these integral seed flours. Moreover, it can assist in formulating nutritional recommendations to promote the incorporation of these natural foods into di ets, given their role as rich sources of bioactive compounds.