Serviços Personalizados
Journal
Artigo
Indicadores
-
Citado por SciELO
Links relacionados
-
Similares em SciELO
Compartilhar
Revista argentina de cardiología
versão On-line ISSN 1850-3748
Rev. argent. cardiol. vol.92 no.1 Ciudad Autónoma de Buenos Aires mar. 2024 Epub 28-Fev-2024
http://dx.doi.org/10.7775/rac.es.v92.i1.20726
ORIGINAL ARTICLE
Administration of N-acetylcysteine Attenuates Post-Myocardial Infarction Remodeling
1 Institute of Cardiovascular Pathophysiology, Department de Pathology, School of Medicine, Buenos Aires University, Argentina
2 Institute of Cardiovascular Pathophysiology (secondary office of the School of Veterinary Sciences), School of Veterinary Sciences, Buenos Aires University, Argentina.
3 Specialty in Veterinary Clinical Cardiology, School of Veterinary Sciences, Buenos Aires University, Argentina.
4 Hospital de Clínicas José de San Martín, Cardiac Surgery Division, School of Medicine, Buenos Aires University, Argentina.
5 Experimental Pathology Center, Department of Pathology, School of Medicine, Buenos Aires University, Argentina.
6 Institute of Cardiovascular Pathophysiology (secondary office of the School of Veterinary Sciences), School of Veterinary Sciences, Buenos Aires University, Argentina.
Background:
Ischemic heart disease is the main cause of death in Argentina. The prevailing form of presentation is myocardial infarction (MI), characterized by insufficient blood perfusion, cell death and loss of contractile mass. The evolution of ventricular remodeling causes ventricular dilation, hemodynamic impairment, heart failure, and decreased survival. The oxidative stress occurring during this process could be attenuated by the administration of N-acetyl cysteine (NAC) through increased glutathione levels.
Objectives:
The aim of this study was to evaluate the effect of NAC administration on post-MI remodeling.
Methods:
New Zealand rabbits were divided into three experimental groups: Control, MI and MI+NAC. A left thoracotomy was performed under general anesthesia, and in the MI groups a branch of the left coronary artery was ligated. Echocardiographic, hemodynamic and morphological studies of the left ventricle were performed at the end of the 28-day post infarction follow-up period.
Results:
In the MI and MI+NAC groups, the infarcts were located in the left ventricular free wall and had similar sizes. The administration of NAC prevented non-infarcted area thinning and decreased the dilation caused by the infarction. It also preserved left ventricular systolic and diastolic functions, attenuating the impairment that they suffered as a consequence of the infarction.
Conclusion:
These results suggest that NAC administration is a promising therapy to mitigate the unfavorable effects of post-MI remodeling.
Key words: N-acetylcysteine; Myocardial infarction; Remodeling
INTRODUCTION
Ischemic heart disease is the main cause of death in high and mid-income countries, such as Argentina. 1 This entity involves several clinical-pathological conditions, myocardial infarction (MI) being the most important one. 2),(3 This condition takes place when blood perfusion is insufficient to maintain the viability of a myocardial area causing its death, with the consequent loss of contractile mass. 4),(5 Additionally, when the infarct size exceeds a certain threshold, a series of structural changes are produced in the walls and ventricular chambers, known as post-MI ventricular remodeling. 6),(7),(8),(9 The evolution of this process slowly and progressively generates ventricular dilation, hemodynamic impairment, heart failure and decreased survival during months and even years. 9),(10),(11),(12 This unfavorable evolution occurs in approximately one third of patients, and it is essential to develop and employ therapeutic measures than can prevent or mitigate it.
Myocardial oxidative stress increases during post-MI cardiac remodeling and in heart failure due to greater generation of oxygen-derived free radicals [reactive oxygen species (ROS)]. 13 Oxidative stress can be defined as the imbalance between oxidant and antioxidant species in favor of the former. In hearts with MI, this redox imbalance damages cardiac myocyte viability and favors hypertrophy which evolves towards a decompensated phenotype. 14 Glutathione (GSH) is a crucial agent in the antioxidant system of the organism and in redox metabolism, and is one of the main antioxidant defenses. 15 It is synthetized in two stages depending on the availability of substrate and regulatory mechanisms. The regulation step in its synthesis takes place at the level of the gamma-glutamyl-cysteinyl synthase enzyme, and cysteine is the limiting amino acid in the process. 16),(17 N-acetyl cysteine (NAC) is a precursor of cysteine that can be administered orally or intravenously to increase the levels of cysteine when GSH concentrations need to be enhanced to favor detoxification processes. Initial clinical studies have demonstrated the efficacy of NAC therapy in the treatment of ischemic heart disease, with mild secondary effects. 18),(19 NAC administration after MI has been efficient to reduce cardiac sympathetic hyperactivity, arrhythmic episodes and infarct size in dogs and rats. 20),(21 Recent studies have started to evaluate NAC efficacy to control post-MI remodeling. In a pilot clinical trial, patients treated with NAC presented less adverse major cardiac events than those treated with placebo. 22 In another study, use of NAC in patients with congestive heart failure has been promising by facilitating the effects of isosorbide dinitrate and reducing nitroglycerine tolerance. 23
Based on these considerations, the present study was designed to improve the understanding of the mechanisms involved in the process of post-MI left ventricular remodeling and to evaluate the possible use of an antioxidant therapy with NAC to relieve the unfavorable events.
METHODS
Experimental model
An experimental model of MI in rabbits was used following the “Guide for the Care and Use of Laboratory Animals” developed by the National Academy of Sciences of the United States of America, updated by the American Physiological Society, 24 and in agreement with the Institutional Committee for the Care and Use of Laboratory Animals (CICUAL) of the Buenos Aires University School of Medicine(Resolution CD 2375/18). To this end, male New Zealand rabbits weighing 2.8 to 3.2 kg, free from infectious and parasitic diseases and which did not present pathological dysrhythmias or echocardiographic abnormalities were included in the study. Following anesthesia induced by intramuscular ketamine (45 mg/kg) and xylazine (0,5 mg/kg), the animals were mechanically ventilated through an endotracheal tube with ambient air using a Harvard respirator (tidal volume 25 ml, respiratory frequency 32-36 cycles/min). A flexible catheter was immediately placed in the marginal vein of the ear and 5% dextrose was infused at 3 ml/min. Using this same venous access, anesthesia was maintained through additional doses of sodium thiopental according to surgical requirements. Electrocardiographic monitoring was carried out during surgery. A left lateral thoracotomy was performed through the fourth intercostal space to expose the heart, and following pericardiectomy a 5.0 silk suture was passed under a prominent branch of the left coronary artery which was ligated to induce MI. Ischemia was confirmed by regional pallor of the cardiac surface. Next, the thoracotomy was closed respecting the different anatomical planes. Antibiotic therapy was then administered (ciprofloxacin, prophylactically) and the animals recovered under analgesia (tramadol 4-5 mg/kg) which was prolonged up to 48 h postoperatively. Animals were housed in specially conditioned cages for 28 days, in a quiet and acclimatized environment, and fed ad libitum a balanced commercial diet and water.
Experimental groups
The following experimental groups were defined:
Group 1 (n=10). In this group surgery was performed using the technique described, but no infarction was induced, as the coronary artery was not ligated (Control group). The animals were allowed their natural evolution for 28 days.
Group 2 (n=9) In this group, MI was induced using the technique described above. The animals were allowed their natural evolution until completing the same period of time as group 1 (MI group).
Group 3 (n=8) In this group, MI was produced by the technique described above and N-acetylcysteine was orally administered at doses of 300 mg/kg/day (MI+NAC group) for 28 days.
Once the evolution period was concluded echocardiographic, hemodynamic, and morphological studies were performed.
Echocardiographic studies
At the end of each experimental protocol, echocardiographic studies were performed under mild anesthesia with midazolam (2 mg/kg). A Esaote MyLab ultrasound machine was used, equipped with 4 to 9 MHz micro convex probe. The following left ventricular parameters were measured: interventricular septal thickness, diastolic and systolic diameters, percent fractional shortening and ejection fraction.
Hemodynamic studies
To assess remodeling and cardiac function from a hemodynamic viewpoint, the animals were anesthetized and a catheter was introduced in the right carotid artery connected to a Hewlett Packard 54S pressure transducer and hemodynamic monitoring equipment, to measure arterial pressure. Then, the catheter was advanced to enter the left ventricle (LV) to evaluate left ventricular hemodynamic behavior, with special reference to end-diastolic pressure (EDP).
Morphological studies
After hemodynamic data were obtained, the animals were euthanized with an intravenous lethal dose of sodium pentobarbital and diphenylhydantoin sodium (Euthanyle ™). The heart was immediately removed, and after macroscopic evaluation, it was dissected, and the infarct scar was weighed as estimation of infarct size.
Statistical analysis
Numerical data are expressed as arithmetic mean and standard error of the mean, and were analyzed using a statistical analysis software package (GraphPad Prism, GraphPad Software, SanDiego, California, USA). Analysis of variance followed by Tukey’s test for multiple comparisons was used for tests of statistical significance. A p value < 0.05 was considered statistically significant.
RESULTS
Infarct size assessment confirmed its absence in the Control group, while the MI and MI+NAC groups presented similar infarct sizes (20.5±0.98 g and 18.50±1.39 g, respectively, p=NS) in the left ventricular free wall. Echocardiographic analysis revealed that the end-diastolic thickness of the interventricular septum, that is, in a remote zone from the infarction was 2.93±0.12 mm in the Control group, 2.36±0.15 mm in the MI group (p < 0.05 vs. the Control group) and 3.00±0.21 mm in the MI+NAC group (p < 0.05 vs. the MI group). At endsystole, the values were 4.68±0.13 mm, 3.84±0.21 mm and 4.71±0.39 mm, respectively. Thus, NAC administration preserved the morphology of the noninfarcted zone, and avoided the thinning produced as part of post-MI remodeling (Figure 1). Regarding left ventricular diameters, the diastolic diameter was 12.94±0.64 mm while the systolic diameter was 8.35±0.50 mm in the Control group. Both diameters were increased in the MI group (20.21±1.14 mm and 14.71±0.77 mm respectively, p < 0.05 vs. Control), while these increases were attenuated in MI+NAC group (16.76±1.00 mm and 12.06±0.81 mm, respectively, p < 0.05 vs. MI) (Figure 2). This indicates that NAC administration decreased the dilation provoked by the infarction.
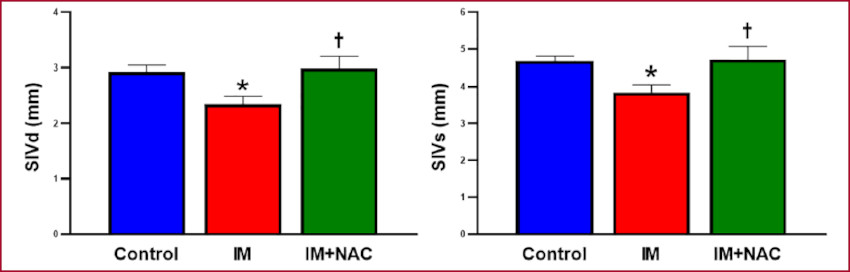
Fig. 1 Interventricular septal thickness in diastole (IVSd) and systole (IVSs). MI: Myocardial infarction; NAC: N-acetylcysteine. *: p < 0.05 vs. Control; †: p < 0.05 vs. MI
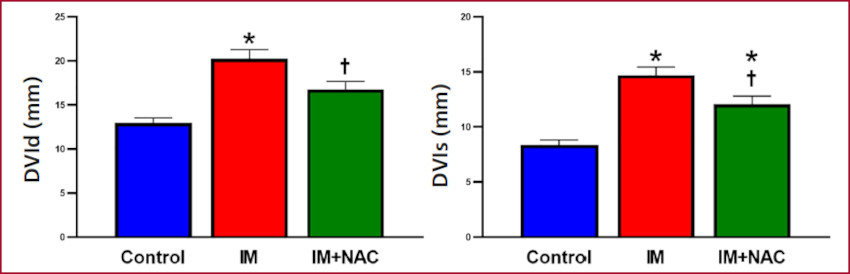
Fig. 2 Left ventricular diastolic and systolic diameters (LVDd and LVDs, respectively) MI: Myocardial infarction; NAC: N-acetylcysteine. *: p < 0.05 vs. Control; †: p < 0.05 vs. MI
Taken together, the preservation of septal thickness and the decreased dilation observed with NAC administration indicate a protective effect of NAC against the adverse remodeling caused by MI.
Fractional shortening was 36.54±1.86% in the Control group; it decreased in the MI group (22.44±1.47%, p < 0.05 vs. Control), while this decrease was attenuated in the MI+NAC group (30.05±2.27%, p < 0.05 vs MI). Similar results were obtained when left ventricular ejection fraction was considered: 68.10±2.43% in the Control group, 47.44±2.81% in the MI group (p < 0.05 vs Control) and 59.20±2.31% in the MI+NAC group (p < 0.05 vs. MI). Therefore, NAC administration in the group with MI, preserved left ventricular function (Figure 3).
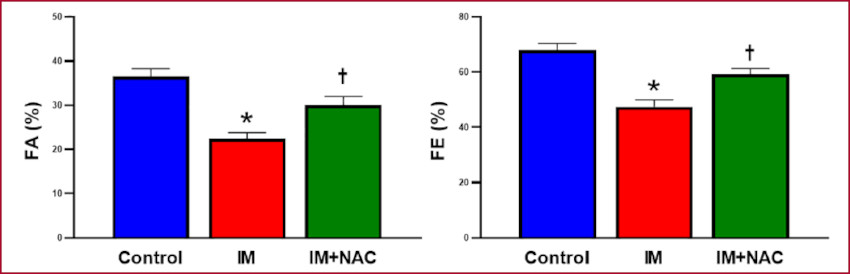
Fig. 3 Percent left ventricular fractional shortening (FS) and ejection fraction (EF). MI: Myocardial infarction; NAC: N-acetylcysteine. *: p < 0.05 vs. Control; †: p < 0.05 vs. MI
Hemodynamic state assessment through arterial pressure showed no differences among the study groups (Figure 4). Conversely, the hemodynamic behavior of the LV showed a clear increase of EDP in the MI group (9.13±1.12 mmHg) compared with the Control group (2.67±0.41mmHg, p < 0.05), which was mitigated by NAC (3.67±1.02 mmHg, p < 0.05 vs. MI) (Figure 5). These results suggest that NAC administration after MI preserves left ventricular diastolic function, attenuating the damage caused by the infarction.
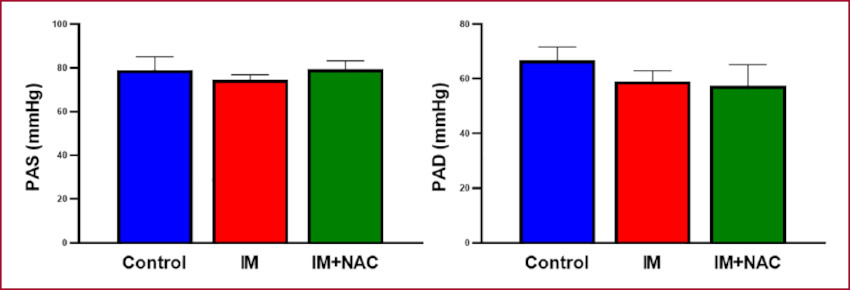
Fig. 4 MI: Myocardial infarction; NAC: N-acetylcysteine. Systolic and diastolic blood pressure (SBP and DBP, respectively)
DISCUSSION
The presence of MI generates a reduction of myocardial contractility as a consequence of loss of functional myocardial mass, but once this acute period is overcome, contractility may continue to decrease due to remodeling and progressive left ventricular dysfunction. The present study used an experimental rabbit model of 28-day evolution myocardial infarction, in which echocardiographic and hemodynamic studies were performed to evaluate the ventricular post-MI remodeling process and the possible use of NAC antioxidant therapy to mitigate its unfavorable effects. These experiments showed a beneficial effect of NAC on the morphology of the non-infarcted area and on ventricular function.
It was possible to observe the preservation of the infarcted area morphology and less post-MI remodeling in the non-infarcted zone, evidencing lower eccentric hypertrophy. Prior studies have shown that MI produces volume overload in the spared sectors, generating cardiomyocyte remodeling with formation of new sarcomeres, among other modifications. 25 The new sarcomeres are positioned in series with the already existing ones, giving rise to greater myocyte longitudinal diameter, which, in turn, generates increased left ventricular chamber volume. It is a peculiar form of eccentric hypertrophy, as it occurs only in the spared territories. 26),(27 Although sarcomere addition could be considered as an adaptive response to balance the loss of contractile mass produced by the infarction, it generates a concomitant enlargement of the ventricular chamber, and hence, of ventricular volume, which by increasing wall stress and consequently, myocardial oxygen consumption, can be deleterious.
It is usual to associate an increase in ventricular volume with greater intraventricular pressure, but this is not necessarily so, as disorders that hamper ventricular filling without modifying chamber volume can also provoke an increase in intraventricular pressure. Therefore, for a more rigorous assessment, we performed a left ventricular hemodynamic study, which showed that end-diastolic intraventricular pressure was increased after MI.
Accumulated evidence indicates that the NADPH oxidase family is the main source of ROS in several cardiovascular diseases, and that NAC is able to act as antioxidant, resulting in reduced oxidative stress. 28 The antioxidant effect of NAC implies a direct interaction with ROS, 29),(30 or a positive regulation of cysteine and GSH biosynthesis. 31),(32 This last compound has been reported to be decreased in hearts with MI. 32),(33 Recent experimental studies in animals and clinical studies have pointed out that the existence of oxidative stress caused by greater production of free radicals would play a key role in post-MI cardiac remodeling, both in early as late stages. 13 Therefore, use of antioxidant therapies after MI is a developing field of research, in which NAC is especially attractive. In this respect, the combined streptokinase and NAC administration in patients with acute myocardial infarction has been shown to decrease oxidative stress and improve ventricular function. 34 N-acetylcysteine, acting as antioxidant, was also able to reverse inflammation and oxidative stress 35 and of preserving connexin 43 in rat models of MI. 36 Beneficial effects of NAC on inflammation were also observed in patients using serum values of transforming growth factor beta (TGF-α) and tumor necrosis factor alpha (TNF-α) as indicators.37 The efficacy of NAC to control post- MI remodeling evaluated in a prospective, double, blind, randomized test clinical trial showed that, compared with a placebo-treated group, patients treated with NAC presented less major adverse cardiac events (including reinfarction) during a oneyear follow-up. 22
Consistently with these results, the assessment of ventricular diameters in our study revealed that NAC administration is able to reduce ventricular dilation produced by MI. The complementary evaluation of left ventricular hemodynamic behavior showed that NAC administration is also able to attenuate the increase of EDP caused by the infarction. Taken together, these results suggest that NAC administration after infarction preserves left ventricular diastolic function, by attenuating the damage elicited by remodeling. Concomitantly, the echocardiographic evaluation showed that NAC administration also attenuates left ventricular ejection fraction impairment.
The results of the present study indicate that NAC administration favorably modifies the initial stages of post-MI remodeling, has a protective effect on the non-infarcted area, decreases dilation and increased EDP and attenuates ejection fraction impairment. Thus, although further studies are necessary to broaden this knowledge, our results suggest that NAC administration is a promising therapy to mitigate the unfavorable effects of post-MI remodeling.
REFERENCES
1. https://www.who.int/es/news-room/fact-sheets/detail/the-top-10-causes-of-death. https://www.who.int/es/news-room/fact-sheets/detail/the-top-10-causes-of-death [ Links ]
2. Kumar V, Abbas AK, Aster JC. Robbins y Cotran. Patología estructural y funcional, 10a ed., Elsevier, 2021. [ Links ]
3. Braunwald E. Heart disease: A textbook of cardiovascular medicine, 4th edition, W. B. Saunders Company, 1992, p.1200. [ Links ]
4. Miura T, Yellon DM, Downey JM. Myocardial infarction. En: Physiology and pathophysiology of the heart, 2nd edition. N. Sperelakis (ed.), Kluwer Academic Publishers, 1989. https://doi.org/10.1007/978-1-4613-0873-7_47 [ Links ]
5. Fishbein M, Maclean D, Maroko P. The histopathologic evolution of myocardial infarction. Chest 1978;73:843-9. https://doi.org/10.1378/chest.73.6.843 [ Links ]
6. Roberts CS, Maclean D, Maroko P, Kloner RA. Early and late remodeling of the left ventricle after myocardial infarction. Am J Cardiol 1984;54:407-10. https://doi.org/10.1016/0002-9149(84)90206-6 [ Links ]
7. Weisman HF, Bush DE, Mannisi JA, Healy Bulkley B. Global cardiac remodeling after acute myocardial infarction: A study in the rat model. J Am Coll Cardiol 1985;5: 1355-62. https://doi.org/10.1016/S0735-1097(85)80348-X [ Links ]
8. Lamas GA, Pfeffer MA. Increased left ventricular volume following myocardial infarction in man. Am Heart J 1986;111:30-5. https://doi.org/10.1016/0002-8703(86)90549-1 [ Links ]
9. Leancă SA, Crișu D, Petriș AO, Afrăsânie I, Genes A, Costache AD, Tesloianu DN, Costache II. Left Ventricular Remodeling after Myocardial Infarction: From Physiopathology to Treatment. Life (Basel) 2022;12:1111. https://doi.org/10.3390/life12081111 [ Links ]
10. Pfeffer MA, Braunwald E. Ventricular remodeling after myocardial infarction. Experimental observations and clinical implications. Circulation 1990;81:1161-72. https://doi.org/10.1161/01.CIR.81.4.1161 [ Links ]
11. Pfeffer JM, Pfeffer MA, Fletcher PJ, Braunwald E. Progressive ventricular remodeling in rat with myocardial infarction. Am J Physiol 1991;260:H1406-14. https://doi.org/10.1152/ajpheart.1991.260.5.H1406 [ Links ]
12. Gaudron P, Eilles C, Kugler I, Ertl G. Progressive left ventricular dysfunction and remodeling after myocardial infarction. Potential mechanisms and early predictors. Circulation 1993;87:755-63. https://doi.org/10.1161/01.CIR.87.3.755 [ Links ]
13. Tsutsui H, Kinugawa S, Matsushima S. Oxidative stress and heart failure. Am J Physiol Heart Circ Physiol 2011;301:H2181-90. https://doi.org/10.1152/ajpheart.00554.2011 [ Links ]
14. Li W, Kennedy D, Shao Z, Wang X, Kamdar AK, Weber M, et al. Paraoxonase 2 prevents the development of heart failure. Free Radic Biol Med 2018;121:117-26. https://doi.org/10.1016/j.freeradbiomed.2018.04.583 [ Links ]
15. Jones D, Carlson JL, Mody VC, Cai J, Lynn M, Sternberg P. Redox state of glutathione in human plasma. Free Rad Biol Med 2000;28:625-35. https://doi.org/10.1016/S0891-5849(99)00275-0 [ Links ]
16. Cho S, Hazama M, Urata Y, Goto S, Horiuchi S, Sumikawa K, et al. Protective rol of glutathione synthesis in response to oxidized low density lipoprotein in human vascular endothelial cells. Free Rad Biol Med 1999;26:589-602. https://doi.org/10.1016/S0891-5849(98)00232-9 [ Links ]
17. Griffith OW. Biological and pharmacologic regulation of mammalian glutathione synthesis. Free Rad Biol Med 1999;27:922-35. https://doi.org/10.1016/S0891-5849(99)00176-8 [ Links ]
18. Ardissino D, Merlini PA, Savonitto S, Demicheli G, Zanini P, Bertocchi F, et al. Effect of transdermal nitroglycerin or N-acetylcysteine, or both, in the long-term treatment of unstable angina pectoris. J Am Coll Cardiol 1997;29:941-47. https://doi.org/10.1016/S0735-1097(97)00005-3 [ Links ]
19. Horowitz JD, Henry CA, Syrjanen ML, Louis WJ, Fish D, Smith TW, et al. Combined use of nitroglycerin and N-acetylcysteine in the management of unstable angina pectoris. Circulation 1988;77:787- 94. https://doi.org/10.1161/01.CIR.77.4.787 [ Links ]
20. Šochman J, Kolc J, Vrána M, Fabián J. Cardioprotective effects of N-acetylcysteine: the reduction in the extent of infarction and occurrence of reperfusion arrhythmias in the dog. Int J Cardiol 1990;28:191-96. Https://doi.org/https://doi.org/10.1016/0167-5273(90)90060-I [ Links ]
21. Lee T-M, Lai P-Y, Chang N-C. Effect of N-acetylcysteine on sympathetic hyperinnervation in post-infarcted rat hearts. Cardiovasc Res 2010;85:137-46. https://doi.org/10.1093/cvr/cvp286 [ Links ]
22. Talasaz AH, Khalili H, Fahimi F, Jenab Y, Broumand MA, Salarifar M, Darabi F. Effects of N-acetylcysteine on the cardiac remodeling biomarkers and major adverse events following acute myocardial infarction: a randomized clinical trial. Am J Cardiovasc Drugs 2014;14:51-6. https://doi.org/10.1007/s40256-013-0048-x [ Links ]
23. Mehra A, Shotan A, Ostrzega E, Hsueh W, Vasquez-Johnson J, Elkayam U. Potentiation of isosorbide dinitrate effects with Nacetylcysteine in patients with chronic heart failure. Circulation 1994;89:2595-600. https://doi.org/10.1161/01.CIR.89.6.2595 [ Links ]
24. https://www.physiology.org/career/policy-advocacy/animalresearch?SSO=Y [ Links ]
25. Anversa P, Ricci R, Olivetti G. Quantitative structural analysis of the myocardium during physiologic growth and induced cardiac hypertrophy: a review. J Am Coll Cardiol 1986;7:1140-49. https://doi.org/10.1016/S0735-1097(86)80236-4 [ Links ]
26. Anversa P, Beghi C, Kikkawa Y, Olivetti G. Myocardial infarction in rats: Infarct size, myocite hypertrophy and capillary growth. Circ Res 1986; 58:26-37. https://doi.org/10.1161/01.RES.58.1.26 [ Links ]
27. Rubin SA, Fishbein MC, Swan HJC. Compensatory hypertrophy in the heart after myocardial infarction in the rat. J Am Coll Cardiol 1983;1:1435-41. https://doi.org/10.1016/S0735-1097(83)80046-1 [ Links ]
28. Okazaki T, Otani H, Shimazu T, Yoshioka K, Fujita M, Iwasaka T. Ascorbic acid and N-acetyl cysteine prevent uncoupling of nitric oxide synthase and increase tolerance to ischemia/reperfusion injury in diabetic rat heart. Free Radic Res 2011;45:1173-83. https://doi.org/10.3109/10715762.2011.605361 [ Links ]
29. Kamunde C, Sharaf M, MacDonald N. H2O2metabolism in liver and heart mitochondria: low emitting-high scavenging and high emitting-low scavenging systems. Free Radic Biol Med 2018;124:135- 48. https://doi.org/10.1016/j.freeradbiomed.2018.05.064 [ Links ]
30. Lu J, Cheng K, Zhang B, Xu H, Cao Y, Guo F, et al. Novel mechanisms for superoxide-scavenging activity of human manganese superoxide dismutase determined by the K68 key acetylation site. Free Radic Biol Med 2015;85:114-26. https://doi.org/10.1016/j.freeradbiomed.2015.04.011 [ Links ]
31. Hill MF, Singal PK. Antioxidant and oxidative stress changes during heart failure subsequent to myocardial infarction in rats. Am J Pathol 1996;148:291-300. [ Links ]
32. Giustarini D, Galvagni F, Dalle Donne I, Milzani A, Severi FM, Santucci A, et al. N-acetylcysteine ethyl ester as GSH enhancer in human primary endothelial cells: a comparative study with other drugs. Free Radic Biol Med 2018;126:202-09. https://doi.org/10.1016/j.freeradbiomed.2018.08.013 [ Links ]
33. Khaper N, Kaur K, Li T, Farahmand F, Singal PK. Antioxidant enzyme gene expression in congestive heart failure following myocardial infarction. Mol Cell Biochem 2003;251:9-15. https://doi.org/10.1023/A:1025448908694 [ Links ]
34. Arstall MA, Yang J, Stafford I, Betts WH, Horowitz JD. N-acetylcysteine in combination with nitroglycerin and streptokinase for the treatment of evolving acute myocardial infarction. Safety and biochemical effects. Circulation 1995;92:2855-62. https://doi.org/10.1161/01.CIR.92.10.2855 [ Links ]
35. Khanna AK, Xu J, Mehra MR. Antioxidant N-acetyl cysteine reverses cigarette smoke-induced myocardial infarction by inhibiting inflammation and oxidative stress in a rat model. Lab Invest. 2012;92:224-35. https://doi.org/10.1038/labinvest.2011.146 [ Links ]
36. Lee TM, Lin SZ, Chang NC. Both PKA and Epac pathways mediate N-acetylcysteine-induced Connexin43 preservation in rats with myocardial infarction. PLoS One 2013;8:e71878. https://doi.org/10.1371/journal.pone.0071878 [ Links ]
37. Talasaz AH, Khalili H, Jenab Y, Salarifar M, Broumand MA, Darabi F. N-Acetylcysteine effects on transforming growth factor-ß and tumor necrosis factor-a serum levels as pro-fibrotic and inflammatory biomarkers in patients following ST-segment elevation myocardial infarction. Drugs R D 2013;13:199-205. https://doi.org/10.1007/s40268-013-0025-5 [ Links ]
Received: October 27, 2023; Accepted: January 25, 2024